Key Considerations For Developing Next-Generation Antibody-Drug Conjugates
By Hyun Yong Cho and Byeong Sung Lee, PinotBio, Inc.
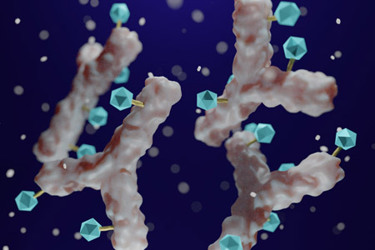
Antibody-drug conjugates (ADCs) are increasingly entering the oncologists' armamentarium. As of the beginning of 2023, 14 different ADCs had been approved globally for various cancer indications.1 These highly complex molecules consist of the optimal balance between target, antibody, linker chemistry, and payload.
The industry's understanding of the best methods to develop ADCs is still evolving. But while these targeted therapies may now be coming to the market, novel formats are already chasing them into the clinical pipeline.2 The proposed advantage of novel format conjugates, compared to more traditional ADCs, is to achieve greater selective localization at the target site, thus increasing the therapeutic index of the payload.2 These approaches include conjugating or fusing payloads to antibodies and antibody alternatives as targeting moieties.
Antibodies have excellent recognition properties that have proven critical in immuno-oncology approaches. Newer formats, such as peptides, protein scaffolds, and aptamers, offer similar target recognition but have been specifically engineered to allow access to new targets, increased control in drug:antibody ratio (DAR), and reduced immunogenicity, for improved effectiveness in drug development.3 Since the approval of the first ADC, many lessons have been learned in ADC development that can be translated to the next wave of these drugs, with additional lessons from antibody alternative development that can be heeded to speed future advances for the benefit of patients.
Complementing Target With Antibody Subtype In ADC Development
Selecting the specific antibody subtype for antibody therapeutics is critical to ensuring maximal therapeutic activity and minimal side effects. Anti-tumor antibody drugs exert therapeutic efficacy by several different, but not mutually exclusive, mechanisms, including (1) Fc-mediated effector functions; (2) blocking tumor growth signals; (3) inhibiting angiogenesis; (4) triggering apoptotic pathways in tumor cells; and (5) activating immune cells.4
Though no stringent criteria exist for selecting antibody subtypes according to target, the target's cellular distribution and biological function should be considered. IgG1 and IgG2 subtypes stimulate FcRγ activity resulting in antibody-dependent cell cytotoxicity (ADCC) or antibody-dependent cell phagocytosis (ADPC).4 In contrast, IgG4 is a poor inducer of FcγR-mediated effector functions.4 Selection of the appropriate antibody can thus create different modes of action that should be matched with the disease biology under treatment.
The well-described mechanism of action for ADC drugs includes (1) binding of the mAb to the target in tumor cells; (2) internalizing the ADC into the cells through receptor-mediated endocytosis; and (3) releasing the cytotoxic payload from the internalized ADC to kill the target cells. While the primary effect of ADCs is mediated via the toxic payload delivered to the cells, Fc-mediated functions can also contribute to their function.4
To date, the majority of ADCs have utilized IgG1 antibodies that exhibit FcγR function (Table 1). Adcetris, for the treatment of CD30-positive lymphoma, is composed of brentuximab IgG1 with a modified auristatin payload. Reports show that this ADC can induce ADCP through its FcγR effects, contributing to its potent anti-tumor effects.5,6 However, some studies have identified Fc- receptor engagement by ADCs as causative of side effects. Kadcyla, an ADC used in the treatment of metastatic breast cancer and composed of trastuzumab covalently linked to the cytotoxic agent DM1, has been shown to internalize into megakaryocytes in vivo via FcγRIIA engagement, causing thrombocytopenia.7,8
During the production of ADCs, payload conjugation often increases the hydrophobicity of the antibody molecule, leading to reduced stability and increased aggregation.22 ADC aggregates are known to enhance the off-target cytotoxicity, but use of a FcγR-blocking antibody or Fc-engineering for silencing Fc-mediated effector functions has the potential to reduce this effect.22 Thus FcγRs play an important role in the internalization of ADC aggregates and increase the potential for off-target toxicity.
Incorporating lessons on target biology and ligand function is critical when developing the new format ADCs that use antibody alternatives. Antibody alternatives, such as bicycles,23 centyrins, 24 and Optimer binders,25 which are being developed as delivery vehicles for novel format ADCs,2 do not possess an Fc region and so lack the ability to activate Fc-mediated functions. For many ADCs, this may be seen as a benefit as there is potential for reduced off-target interactions. However, where ADCC and ADPC effects promote the activity of the ADC, FcγR activating antibodies remain a vital part of the ADC developer's toolkit.
Optimizing Format For Half-life Functionality
The pharmacokinetic and pharmacodynamic properties of ADCs, such as their in vivo half-life, depend in part on the affinity ligand format used. For standard IgG antibodies, the half-life of IgG1, IgG2, and IgG4 subtypes is approximately 21 days in serum.26 For IgG3, this is reduced to approximately seven days.26 For the approved ADCs, the average half-life of these molecules is seven days in vivo (Table 1). This stability allows these drugs to circulate for sufficient time to reach their target tissue before being eliminated and provides an increased half-life over the cytotoxin alone, which would be rapidly cleared through renal filtration due to the small molecular weight.27
While this long half-life has been seen as desirable, overcoming the need for repeated patient dosing, complications include the large molecular weight, which reduces extravasation across capillary walls and diffusion into solid tumor masses.27 As the majority of ADCs are prepared using thiol-maleimide chemistry, they can undergo deconjugation of the drug linker from the antibody, particularly for linkers containing a maleimidocaproyl moiety. In these cases, as much as 50%-75% of the drug linker is deconjugated in plasma over ~seven days. The deconjugated drug linker reacts with thiol-containing plasma molecules such as albumin, potentially contributing to toxicities by increasing payload half-life and off-target effects.27,28 At PinotBio, we use our Pinot-ADC platform to provide a range of drug-linker systems for compatibility with different release strategies, including a pH-sensitive linker that allows rapid and highly effective drug release intracellularly and within the tumor microenvironment (Fig 1). Pinot-ADC also uses a new payload that has been shown to be safe when administered alone, even at relatively high doses in non-human primate toxicological studies and allows a wide therapeutic window.29
PinotBio is developing this drug linker system with both antibodies for ADC generation and novel affinity formats in Optimer binders for novel format ADCs.30,31 Optimer binders are affinity ligands that can be used as delivery vehicles to target payloads. With a molecular weight of 15-30kDa they offer a smaller molecular size for potential improved extravasation and tissue penetration compared to antibodies.32 Optimer binders are affinity ligands synthesized from DNA or RNA, allowing tuning of the molecular half-life for both hit-and-run strategies or longer residence times.33
Delivering Next-generation Payloads With Diverse MOA
Enhertu from Daichii-Sankyo and Trodelvy from Gilead have shown excellent efficacy in the delivery of topoisomerase I inhibitor payloads. While these results are promising, the problem of resistance to the highly toxic chemotherapeutics delivered by ADCs remains.34 Resistance to topoisomerase I is hypothesized to be due to several possible mechanisms, including:
- reduced topo I expression levels
- mutation in the metabolizing enzyme of structure of topo I
- activation of NF-ĸB, which suppresses cell apoptosis
- reduced intracellular drug accumulation by active drug efflux 34
Following this, the industry is looking for novel ways to advance on these platforms. The FL-118 payload used in Pinot-ADC is a novel camptothecin-derived payload that offers comparative performance to other camptothecin analogues in the inhibition of topoisomerase I. However, FL-118 is also able to selectively inhibit the expression of multiple members of the inhibitor of apoptosis family and the Bcl-2 family as a secondary mode of action (Fig 1).35, 36 Additionally, as FL-118 bypasses the drug resistance induced by multiple ABC transporter efflux proteins,37,38 it remains effective for the treatment of tumors that acquire resistance to earlier generation camptothecins such as irinotecan and topotecan.39
Figure 1: Pinot-ADC utilizes multiple mechanisms of action to help overcome resistance of earlier generation drugs, with a novel linker system.29 Click on image to enlarge.
ADCs are beginning to deliver on the promise of new precision therapies for cancer treatment. From the first approval of an ADC in 2017 to today’s expanding pipeline, the industry has worked to develop these molecules and continually refine every aspect: targets, targeting moieties, linkers, and payloads. With each advancement, safety profiles and therapeutic efficacy can be improved, but a central lesson for ADC development is that every aspect of the molecule must be considered in tandem to ensure optimal performance. As the field progresses and more ADCs are brought to market, these incremental enhancements, focusing on balancing the best technologies for each disease indication, will hopefully support improved patient treatments and better patient outcomes in oncology.
References
- Bio-ITWorld.com. FDA Approved ADC Drugs list up to 2022. Nov 2022. Available at: https://www.bio-itworld.com/pressreleases/2022/11/28/fda-approved-adc-drugs-list-up-to-2022
- Beacon Intelligence. The rise of novel format conjugates: A landscape deep dive. Hanson Wade. Oct. 2022
- Cho Hy, Lee BS. ADCs Are The Future Of Precision Chemotherapeutics. Bioprocess Online. 23 Aug 2022. Available at: https://www.bioprocessonline.com/doc/adcs-are-the-future-of-precision-chemotherapeutics-0001
- Yu J, Song Y, Tian W. How to select IgG subclasses in developing anti-tumor therapeutic antibodies. J Haematol Oncol. 2020;13:45.
- Weiskopf K, Weissman IL. Macrophages are critical effectors of antibody therapies for cancer. MAbs. 2015;7(2):303–10.
- Gong Q, Ou Q, Ye S, Lee WP, Cornelius J, Diehl L, et al. Importance of cellular microenvironment and circulatory dynamics in B cell immunotherapy. J Immunol. 2005;174(2):817–26.
- Uppal H, Doudement E, Mahapatra K, Darbonne WC, Bumbaca D, Shen B-Q, et al. Potential mechanisms for thrombocytopenia development with trastuzumab emtansine (T-DM1). Clin Cancer Res. 2015;21(1):123–33.
- Zhao H, Gulesserian S, Ganesan SK, Ou J, Morrison K, Zeng Z, et al. Inhibition of megakaryocyte differentiation by antibody-drug conjugates (ADCs) is mediated by macropinocytosis: implications for ADC-induced thrombocytopenia. Mol Cancer Ther. 2017;16(9):1877–86.
- Food & Drug Administration. Highlights of prescribing information, Enhertu. Available at: https://www.accessdata.fda.gov/drugsatfda_docs/label/2019/761139s000lbl.pdf
- Food & Drug Administration. Highlights of prescribing information, Elahere. Available at: https://www.accessdata.fda.gov/drugsatfda_docs/label/2022/761310s000lbl.pdf
- Food & Drug Administration. Highlights of prescribing information, Tivdak. Available at: https://www.accessdata.fda.gov/drugsatfda_docs/label/2021/761208Orig1s000lbledt.pdf
- European Medicines Agency. Summary of product characteristics, Lumoxiti. Available at: https://www.ema.europa.eu/en/documents/product-information/lumoxiti-epar-product-information_en.pdf
- Food & Drug Administration. Highlights of prescribing information, Besponsa. Available at: https://www.accessdata.fda.gov/drugsatfda_docs/label/2017/761040s000lbl.pdf
- European Medicines Agency. Summary of product characteristics, Kadcyla. Available at: https://www.ema.europa.eu/en/documents/product-information/kadcyla-epar-product-information_en.pdf
- Food & Drug Administration. Highlights of prescribing information, Adcetris. Available at: https://www.accessdata.fda.gov/drugsatfda_docs/label/2014/125388_s056s078lbl.pdf
- Food & Drug Administration. Highlights of prescribing information, Mylotarg. Available at: https://www.accessdata.fda.gov/drugsatfda_docs/label/2006/021174s020lbl.pdf
- Food & Drug Administration. Highlights of prescribing information, Polivy. Available at: https://www.accessdata.fda.gov/drugsatfda_docs/label/2019/761121s000lbl.pdf
- Food & Drug administration. Highlights of prescribing information, Padcev. Available at: https://www.accessdata.fda.gov/drugsatfda_docs/label/2019/761137s000lbl.pdf
- Food & Drug Administration. Highlights of prescribing information, Trodelvy. Available at: https://www.accessdata.fda.gov/drugsatfda_docs/label/2020/761115s000lbl.pdf
- Food & Drug Admnistration. Highlights of prescribing information, Blenrep. Available at: https://www.accessdata.fda.gov/drugsatfda_docs/label/2020/761158s000lbl.pdf
- Food & Drug Administration. Highlights of prescribing information, Zynlonta. Available at: https://www.accessdata.fda.gov/drugsatfda_docs/label/2021/761196s000lbl.pdf
- Aoyama M, Tada M, Yokoo H, Demizu Y, Ishii-Watabe A. Fcγ Receptor-Dependent Internalization and Off-Target Cytotoxicity of Antibody-Drug Conjugate Aggregates. Pharm Res. 2022 Jan;39(1):89-103
- Bicycle Therapeutics. Bicycle Conjugates. Available at: https://www.bicycletherapeutics.com/programs/bicycle-conjugates/
- Aro Biotherapeutics. Pipeline & Programs. Available at: https://www.arobiotx.com/pipeline
- Aptamer Group. Optimer-drug conjugates. Available at: https://aptamergroup.com/wp-content/uploads/2023/02/Optimer®-drug-conjugates.pdf
- Coleman N, Yap TA, Heymach JV, Meric-Burnstam F, Le X. Antibody-drug conjugates in lung cancer: dawn of a new era? NPJ Precis Oncol. 2023 Jan 11;7(1):5.
- Colombo R, Rich JR. The therapeutic window of antibody drug conjugates: A dogma in need of revision. Cancer Cell. 2022 Nov 14;40(11):1255-1263.
- Nilsen J, Bern M, Sand KMK, Grevys A, Dalhus B, Sandlie, I, Andersen JT. Human and mouse albumin bind their respective neonatal Fc receptors differently. Sci. Rep., 8 (2018), Article 14648.
- PinotBio. Available at: https://www.pinotbio.com/
- Koread Biomed. Celltrion signs ADC platform technology contract with Pinot Bio. Oct 2022. Available at: https://www.koreabiomed.com/news/articleView.html?idxno=14828
- Aptamer Group. Aptamer Group and PinotBio extend collaboration for the development of Optimer-drug conjugates. Available at: https://aptamergroup.com/aptamer-group-and-pinotbio-extend-collaboration-for-the-development-of-optimer-drug-conjugates/
- Aptamer Group. From aptamer to Optimer. Available at: https://aptamergroup.com/from-aptamer-to-optimer/
- Aptamer Group. The stability of aptamers. Available at: https://aptamergroup.com/the-stability-of-aptamers/
- Wu ZX, Yang Y, Zeng L, Patel H, Bo L, Lin L, Chen ZS. Esablishment and characterisation of an irinotecan- resistant human colon cancer cell line. Front Oncol. 2021 Feb 22;10:624954.
- Ling X, Cao S, Cheng Q, Keefe JT, Rustum YM, Li F. A novel small molecule FL118 that selectively inhibits survivin, Mcl-1, XIAP and cIAP2 in a p53-independent manner, shows superior antitumor activity. PLoS One. 2012;7:e45571.
- Zhao J, Ling X, Cao S, Liu X, Wan S, Jiang T, Li F. Antitumor activity of FL118, a survivin, Mcl-1, XIAP, and cIAP2 selective inhibitor, is highly dependent on its primary structure and steric configuration. Mol Pharm. 2014;11:457–467.
- Ling X, Liu X, Zhong K, Smith N, Prey J, Li F. FL118, a novel camptothecin analogue, overcomes irinotecan and topotecan resistance in human tumor xenograft models. Am J Transl Res. 2015;7:1765–1781.
- Westover D, Ling X, Lam H, Welch J, Jin C, Gongora C, Del Rio M, Wani M, Li F. FL118, a novel camptothecin derivative, is insensitive to ABCG2 expression and shows improved efficacy in comparison with irinotecan in colon and lung cancer models with ABCG2-induced resistance. Mol Cancer. 2015;14:92.
- Wang J., Liu Z., Zhang D., et al. FL118, a novel survivin inhibitor, wins the battle against drug-resistant and metastatic lung cancers through inhibition of cancer stem cell-like properties. Am J Trans Res. 2017;9(8):3676–3686.
About The Authors:
Hyun Yong Cho is chief scientific officer at PinotBio, Inc. He is in charge of development of next-generation antibody-drug conjugate (ADC) candidates and platforms. He obtained his Ph.D. in biomolecular engineering at the Korea Advanced Institute of Science and Technology. He built up his career throughout all the stages of chemical product commercialization, including fundamental research on organic chemistry-based semiconductor materials, process design, and development at Samsung Cheil Industries (Electronic Materials Division, Samsung SDI, at present) and Heraeus, a German fine chemicals company.
Byeong Sung Lee leads the drug discovery team at PinotBio, Inc. He obtained his Ph.D. in biotechnology at Ajou University, Korea, and then studied bioconjugation at the Molecular Science and Technology Research Center, Ajou University, as a researcher. His research focus is protein engineering and antibody-drug conjugation.
Editor’s Note: Check out our other recent articles on ADCs:
- ADCs Are The Future Of Precision Chemotherapeutics
- 4 Focus Areas To Expand The Success Of Antibody-Drug Conjugates
- The Clinical Landscape Of ADCs In 2023: Diverse Technologies, Narrow Target
- A Payday For Payloads: The Transactional Landscape Of ADCs