How To Overcome Immune Rejection In iPSC-based Gene Therapy
By Jack (Jie) Huang, MD, Ph.D., chief scientist, founder/CEO, CSTEAM biotechnology
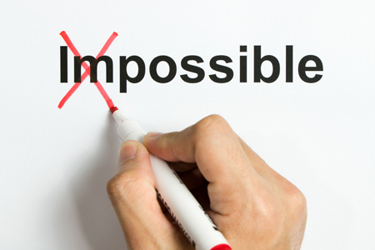
Induced pluripotent stem cells (iPSCs) have revolutionized regenerative medicine and gene therapy, providing a groundbreaking approach to create patient-specific cells that can be genetically corrected and differentiated into virtually any cell type.1 This technology promises transformative applications in a range of diseases, from genetic disorders and neurodegenerative diseases to cardiovascular disease and even cancer (which I discussed in my earlier article here). By using the patient's own cells, iPSCs introduce a personalized and potentially more compatible treatment option, minimizing the risk of immune rejection typically associated with donor-derived stem cells or tissues.2,3 However, despite the enormous advantages, immune rejection remains a significant challenge for iPSC-based therapies.
Interestingly, even when iPSCs are derived from the patient's cells, immune rejection can still occur. This immune response can be triggered by a variety of factors, including epigenetic changes during the reprogramming process, genetic mutations that arise in iPSCs over time, or the unintended expression of immunogenic proteins.4,5 These complications can result in an immune response against the cells intended for therapeutic use. Understanding and addressing these immunogenic factors is therefore critical for the successful integration of iPSCs, especially in therapies that require long-term survival and functional integration into host tissues, such as cardiac, liver, or neural tissue repair.
To mitigate immune rejection, researchers are exploring several strategies. One approach is to genetically engineer iPSCs to downregulate or knock out immune-activating antigens while retaining their therapeutic function.6 Additionally, immunosuppressive therapy or brief use of immunomodulatory drugs can reduce immune responses after transplantation. Another promising strategy is to engineer iPSCs to match universal “immune evasion” characteristics, using advanced gene editing tools such as CRISPR to selectively alter antigen presentation and immune recognition pathways.7,8
This comprehensive article will explore the causes of immune rejection in iPSC-based therapies, highlighting the need for continued research to overcome these barriers. As iPSC technology advances, improving these strategies will be critical to fully realizing the potential of iPSCs in clinical applications, leading to safer, more effective, and more universally accessible therapies.
Figure 1: Strategies to Overcome Immune Rejection in iPSC-based Therapies
Overcoming immune rejection in iPSC-based therapies involves several approaches. First, optimizing reprogramming techniques can help minimize genetic changes that could trigger an immune response. Removal of immunogenic antigens via CRISPR can reduce immune activation. Immunomodulation using checkpoint inhibitors or regulatory T cells can promote temporary tolerance. Development of universal iPSCs with β2-microglobulin knockout and HLA-E expression addresses compatibility issues. Finally, inducing immune tolerance through strategies such as tolerance-inducing vaccines can adapt the immune system to accept iPSC-derived cells, thereby facilitating safe clinical applications.
Understanding Immune Rejection In iPSC-Based Gene And Cell Therapies
Although iPSCs are theoretically patient-specific, researchers have observed immune rejection in various experimental and preclinical settings.9 There are several major factors that contribute to this unexpected immune rejection, discussed below.
Epigenetic Changes During Reprogramming
iPSCs are generated by reprogramming adult somatic cells to return them to a pluripotent state, allowing them to differentiate into any cell type in the body. This reprogramming process is a complex one that involves resetting the identity of the cell and, in particular, it also requires extensive modifications to the epigenetic landscape.10 Epigenetic modifications, including DNA methylation, histone modifications, and chromatin remodeling, are critical for regulating gene expression in newly generated iPSCs.11 However, during the reprogramming process, these modifications can sometimes lead to the expression of unexpected or aberrant antigens, as these proteins are not normally present in the patient's normal cells. These new antigen expressions can generate immunogenic responses, as the immune system recognizes these iPSC-derived cells as foreign or abnormal.12 For example, proteins associated with early development or non-target cell types may trigger immune surveillance and potential rejection due to aberrant epigenetic changes. In addition, subtle changes in gene expression caused by these reprogramming-induced epigenetic changes also can affect the immune system's interaction with iPSCs, as immune cells may perceive these altered expressions as threats.
Therefore, addressing the immunogenicity issues caused by epigenetic changes in iPSCs is critical for their successful integration into clinical treatments. Researchers are currently exploring ways to identify and regulate these epigenetic shifts to minimize immune responses. For example, improving the reprogramming process to maintain or restore epigenetic patterns that match patients can help reduce antigenicity.13,14 In addition, the use of targeted gene editing tools such as CRISPR/Cas9 to control specific gene expression is also expected to mitigate the immune risks associated with abnormal epigenetic modifications.15
Accumulation of Genetic Mutations
The process of reprogramming somatic cells into iPSCs and their subsequent expansion in culture can result in the accumulation of genetic mutations. These mutations occur as a result of errors in DNA replication, oxidative stress, or prolonged exposure to cell culture conditions.16 Over time, these changes can introduce abnormalities in the genome, leading to the production of altered or new proteins that were not present in the original patient cells. In addition, these new or modified proteins may be recognized as foreign by the immune system, triggering an immune response.17 For example, mutations in key regulatory genes or tumor suppressor genes may result in the expression of abnormal proteins, leading to immune surveillance and potential rejection. This risk is particularly concerning for clinical applications, as immune-mediated destruction of iPSC-derived cells can reduce therapeutic efficacy and may pose safety risks. Therefore, addressing genetic stability issues in iPSC-based therapies involves careful optimization of culture conditions, monitoring mutations through genome sequencing, and potentially using gene editing tools to correct or prevent mutation accumulation. In particular, genetic fidelity throughout the reprogramming and culture process is critical to developing safe, immune-compatible iPSC therapies for clinical applications.
Immunogenicity of Pluripotent Cells
Research has shown that pluripotent cells, such as iPSCs, can inherently express certain immunogenic proteins that are not normally present in adult tissues.18 These proteins often include developmental antigens or other molecules associated with the pluripotent state, such as embryonic antigens. When iPSCs or their derivatives are introduced into a patient, these unusual antigens may be recognized as foreign by the immune system, thereby triggering an immune response. Addressing this immunogenicity requires careful regulation of iPSC gene expression, as well as screening and selection methods to ensure that differentiated cells are less likely to trigger an immune response.
Altered Major Histocompatibility Complex Expression
Major histocompatibility complex (MHC) class I molecules are essential for immune recognition because they present cellular proteins to cytotoxic T cells and help the immune system distinguish between self and non-self. During the reprogramming or culture of iPSCs, the expression of MHC class I molecules may be altered, resulting in reduced or abnormal MHC levels. Such changes not only prevent normal immune recognition but also promote natural killer (NK) cells and other innate immune components to recognize iPSC-derived cells as foreign cells and trigger immune responses. This can cause immune rejection of transplanted cells.19 Therefore, efforts to regulate MHC expression through gene editing or culture optimization can effectively maintain appropriate immune compatibility and reduce the risk of rejection in iPSC-based therapies.
Clinical Examples Of Immune Rejection
In 2011, a key study by Zhao et al. demonstrated that even when autologous cells are used, immune rejection can still occur with iPSC-based therapies.20 In this study, iPSCs from mice were transplanted back into genetically identical (syngeneic) mice, but immune rejection was observed. This finding was unexpected because cells from the same individual are generally considered immune-tolerant, making autologous iPSC-based therapies a promising option to reduce the risk of immune responses.
Researchers attribute this immune rejection to two main factors: epigenetic memory and accumulated mutations. During the reprogramming process, residual epigenetic marks from the original cell type may remain, which can lead to immune recognition of these cells as foreign. In addition, genetic mutations acquired during reprogramming and subsequent cell culture can lead to the expression of altered or new proteins that can trigger an immune attack.21,22
This study highlights the challenges facing iPSC-based therapies, highlighting that immune rejection remains a potential obstacle, even for cells derived from a patient's own tissue. To reduce these risks, researchers are exploring various strategies, including improving reprogramming methods to maintain genetic and epigenetic fidelity and introducing genetic modifications to reduce immunogenicity. This study highlights the importance of further research into developing methods that can prevent immune rejection to improve the clinical feasibility of iPSC-based treatments for a variety of diseases.
Strategies To Overcome Immune Rejection In iPSC-based Gene And Cell Therapy
To realize the full potential of iPSC-based gene and cell therapy, researchers have developed several strategies to minimize its immune rejection. These strategies include optimizing reprogramming methods, using gene editing technologies, modulating immune responses, and developing “universal” iPSCs.
Optimizing Reprogramming and Differentiation Protocols
One way to minimize immune rejection is to improve reprogramming and differentiation protocols to reduce the likelihood of introducing epigenetic abnormalities or genetic mutations. Some key strategies include the following.
Improved Reprogramming Techniques
Initial methods for generating iPSCs often relied on viral vectors that integrated into the host genome to deliver reprogramming factors. While effective, these integration-based methods have raised safety concerns due to the risk of introducing mutations, potentially disrupting critical genes, and increasing immunogenicity. Recent advances in reprogramming technologies aim to reduce these risks through the use of non-integrating vectors such as episomal plasmids, mRNA, small molecules, and recombinant proteins.23–26 These methods facilitate a more controlled reprogramming process without permanently altering the cell's genome, thereby reducing the potential for genetic instability. Non-integrating methods also enable greater control over gene expression levels during the reprogramming process, further minimizing unexpected immune responses. As a result, these new technologies offer a safer and more efficient way to generate iPSCs, increasing their suitability for clinical use in regenerative medicine and gene therapy.
Screening for Genetic and Epigenetic Stability
To improve the safety of iPSC-based therapies, iPSC-derived cells can be thoroughly screened for genetic mutations and epigenetic changes prior to transplantation. Technologies such as whole genome sequencing, RNA sequencing, and methylation profiling allow researchers to detect any genetic instability, unexpected mutations, or epigenetic aberrations that could lead to immunogenicity.27,28 By identifying and selecting stable, non-immunogenic cells for therapy, this rigorous screening process helps ensure that transplanted cells are less likely to elicit an immune response, thereby improving the overall efficacy and safety of the therapy.
Epigenetic Reprogramming
Epigenetic reprogramming technology is essential to improve the immunogenicity and stability of iPSCs, aiming to eliminate abnormal epigenetic marks that may trigger immune rejection. During the reprogramming process, cells can retain certain residual epigenetic marks of their original cell type, which can lead to abnormal gene expression and immune activation upon transplantation. To address this issue, researchers use small molecules and other epigenetic modifiers to reset DNA methylation patterns and histone modifications in iPSCs. By effectively "clearing" these marks, cells can achieve a more realistic pluripotent state, minimize immunogenicity, and enhance compatibility for therapeutic applications.29 This improvement in epigenetic features enables iPSCs to integrate more seamlessly into the host, thereby improving their clinical viability and reducing the risk of immune responses after transplantation.
Gene Editing to Remove Immunogenic Antigens
Advances in gene editing technologies, especially CRISPR-Cas9, have provided powerful tools to remove or modify genes that cause immune rejection. Researchers are exploring the following gene editing approaches.
Removal of Immunogenic Proteins
One innovative strategy is to minimize immune rejection by using the CRISPR-Cas9 gene editing system to selectively remove antigens from iPSC therapies. This approach allows scientists to identify and precisely delete genes responsible for producing proteins or antigens that may trigger an immune response in the recipient.30 For example, the immune system may mark certain proteins associated with pluripotency or abnormal gene expression unique to iPSC-derived cells as foreign, leading to rejection. By using CRISPR-Cas9, researchers can systematically knock out these immunogenic genes in iPSC-derived cells, resulting in more immunocompatible cell products.31 Targeting pluripotency-associated antigens or proteins with abnormal expression patterns reduces the risk of being recognized and attacked by host immune cells. This genetic customization allows scientists to produce iPSCs with more controlled and stable antigenic profiles that are tailored to avoid immune responses while retaining the cells' therapeutic function. With the continuous advancement of CRISPR-Cas9 technology, this precise immunogenic protein removal method shows great promise for optimizing the safety and efficacy of iPSC-based regenerative therapies, especially for long-term integration in clinical applications.
Engineered MHC
To minimize immune rejection, iPSCs can be engineered to express MHC molecules that match the patient's immune profile. In cases where MHC expression is disrupted during the reprogramming process, CRISPR technology can restore normal MHC expression, enhancing compatibility and reducing the risk of immune detection in iPSC-based therapies.32
Immunomodulation
Another approach to overcoming immune rejection is to modulate the patient's immune system to promote tolerance to iPSC-derived cells. Strategies include:
Immunosuppressive Drugs
Traditionally, immunosuppressive drugs, including tacrolimus and cyclosporine, have been used to prevent immune rejection in organ and cell transplants by suppressing the recipient’s immune response. However, long-term use of these drugs carries significant risks, such as increased susceptibility to infection and increased cancer risk, making them less suitable for long-term iPSC-based therapies. To mitigate immune rejection in iPSC transplants without compromising patient safety, researchers have proposed using immunosuppressive drugs for a short period immediately after transplantation.33 This limited approach provides a critical window to help the newly introduced cells integrate and evade immune detection during the initial phase, potentially reducing the need for long-term immunosuppression. This approach aims to strike a balance, providing the protective effects of immunosuppressants to prevent early rejection while minimizing the risks associated with long-term immunosuppression. Ongoing studies are evaluating the optimal dose and duration to maximize the benefits of this approach in clinical iPSC therapies.
Immune Checkpoint Inhibitors
The use of immune checkpoint inhibitors, such as PD-1 and CTLA-4 inhibitors, is a promising strategy to enhance tolerance to iPSC-derived cells. These inhibitors, widely used in cancer immunotherapy, block immune checkpoints that would normally trigger immune recognition and attack. By inhibiting these pathways, checkpoint inhibitors help prevent the immune system from recognizing iPSC-derived cells as foreign. This approach could create a more favorable environment for the survival and function of transplanted cells, potentially minimizing the need for broad immunosuppressive therapies and improving the success of iPSC-based therapies.34,35
Regulatory T cells (Tregs)
Regulatory T cells (Tregs) are critical for maintaining immune tolerance and preventing autoimmune responses, making them a promising tool to support iPSC-based therapies. Increasing the number or activity of Tregs can help suppress immune responses against transplanted iPSC-derived cells. Tregs work by secreting anti-inflammatory cytokines and directly interacting with effector T cells, thereby suppressing immune responses that could lead to rejection. In iPSC transplants, expanding or activating Tregs in recipients can create an immune environment that is more favorable for the integration and survival of these cells, potentially reducing the need for other immunosuppressive measures. Researchers are actively exploring strategies to increase the number or function of Tregs around the site of iPSC-derived cell transplantation to improve the tolerability and long-term efficacy of iPSC therapies.36
Development of Universal iPSCs
One of the most exciting areas of research is the development of “universal” iPSCs that can be used in any patient without triggering immune rejection. This approach involves modifying iPSCs to evade immune detection by targeting MHC genes and other immune-related pathways.
HLA Editing
HLA editing, achieved by knocking out or downregulating human leukocyte antigen (HLA) genes, is a promising strategy to render iPSCs "invisible" to the immune system. HLA class I and II molecules normally present antigens to T cells, thereby triggering an immune response. By removing these molecules, researchers aim to prevent immune recognition of iPSC-derived cells. However, cells lacking HLA are susceptible to attack by NK cells, so additional modifications, such as the introduction of HLA-E, are needed to counteract NK cell-mediated clearance and improve transplant survival.37
Engineered HLA-E Expression
To improve the immunocompatibility of allogeneic iPSC therapies, iPSCs can be engineered to express HLA-E, a nonclassical MHC molecule that helps evade NK cell-mediated lysis.38 HLA-E interacts with inhibitory receptors on NK cells, specifically CD94/NKG2A, favoring the inhibition of NK cell activation and protecting transplanted cells from immune attack. This strategy enables iPSCs to avoid detection by both T and NK cells, as the absence of classical HLA molecules prevents T cell recognition, while HLA-E inhibits NK cell responses. Thus, engineered HLA-E expression in iPSCs creates a more immunocompatible cell line suitable for transplantation between different recipients without the need for extensive immunosuppression. This engineering has important implications for broader clinical applications and is a step toward universal iPSCs for regenerative medicine.
β2-microglobulin Gene Knockout
Knocking out the β2-microglobulin gene, a key component of MHC class I molecules, is a promising strategy to reduce antigen expression in iPSC-derived cells and thereby minimize immune recognition.39 By removing β2-microglobulin, MHC class I molecules become unstable, significantly reducing antigen presentation that may trigger an immune response. When combined with other immune evasion techniques, such as inhibiting NK cell activation by engineering the expression of non-canonical MHC molecules such as HLA-E, this approach could create universal donor cell lines, improve compatibility between recipients, and thereby support safer, broader cell therapy applications.
Tolerance-inducing Strategies
Another innovative approach is to induce immune tolerance by reprogramming the immune system to accept iPSC-derived cells as "self". This can be achieved by the following.
Mixed Chimerism
The creation of mixed chimeras (where donor and recipient cells coexist within the immune system) has emerged as a potential strategy to promote immune tolerance in transplants of iPSC-derived cells. This approach may involve transplanting hematopoietic stem cells (HSCs) from the same donor along with iPSC-derived cells to help the recipient's immune system adapt and tolerate the new cells. By combining donor and recipient cells, mixed chimeras reduce the likelihood of immune rejection, creating a more favorable environment for long-term integration of iPSC-based therapies without the need for long-term immunosuppression. This approach offers a promising avenue for durable cellular tolerance in regenerative medicine.40
Tolerance-inducing Vaccines
Tolerogenic vaccines represent a novel approach to promote immune tolerance in transplants of iPSC-derived cells. These vaccines work by selectively targeting immune responses and encouraging the immune system to tolerate specific antigens present on iPSC-derived cells while maintaining overall immune function to protect against infection and other threats. Such vaccines can be designed to deliver tolerogenic peptides or immunomodulators to suppress T cell activity against iPSC-associated antigens, thereby reducing the risk of rejection. By conditioning the immune system to recognize iPSC-derived cells as "self," tolerogenic vaccines offer a strategic approach to achieve long-term cellular integration and therapeutic effects without extensive immunosuppression, making them a promising tool in regenerative medicine.41,42
Future Directions And Clinical Relevance
As iPSC-based gene and cell therapies move toward clinical use, overcoming immune rejection remains a major challenge. Current strategies, ranging from gene editing to immunomodulation and tolerance induction, are making progress, but a delicate balance is required to maintain immune tolerance without compromising safety. Excessive immunosuppression may expose patients to infection or other complications, so continuous improvement of methods is needed to ensure safe and durable integration of iPSC-derived cells.43
Future directions may involve combination strategies such as gene editing to reduce immunogenicity, HLA engineering to evade T cells and NK cells, and transient use of immunosuppressants to allow early transplant acceptance. In addition, tolerance-inducing vaccines and mixed chimerism technologies hold promise for more precisely adapting transplanted cells to immunity. And continued research into the mechanisms of immune rejection may yield new interventions that ultimately improve the safety and efficacy of iPSC-based therapies and expand their clinical relevance in a range of diseases, including genetic, neurodegenerative, and regenerative diseases.44
Conclusion
Overcoming immune rejection in iPSC-based gene and cell therapies is critical to realizing their full therapeutic potential across a broad range of diseases. Advances in reprogramming technologies have minimized immunogenic changes, while gene editing and immunomodulatory techniques hold promise for fine-tuning cellular properties to evade immune detection. The development of universal immune-compatible cells further supports the feasibility of iPSC-based therapies across diverse patient populations. In addition, ongoing research and carefully monitored clinical trials are helping to refine these strategies to ensure that iPSC-based therapies can be safely, effectively, and broadly applied in personalized medicine. I believe that through these ongoing efforts, iPSC-based therapies may become a cornerstone of regenerative and precision medicine, providing lasting solutions for patients suffering from genetic, degenerative, and complex diseases.
References
- Jonas Cerneckis et al., Induced pluripotent stem cells (iPSCs): molecular mechanisms of induction and applications. Signal Transduction and Targeted Therapy 2024; 9: 112 (https://doi.org/10.1038/s41392-024-01809-0)
- Giulia Paolini Sguazzi et al., Induced Pluripotent Stem Cells (iPSCs) and Gene Therapy: A New Era for the Treatment of Neurological Diseases. International Journal of Molecular Sciences 2021; 22: 13674 (doi: 10.3390/ijms222413674)
- Sangbae Park et al., Engineering considerations of iPSC-based personalized medicine. Biomaterials Research 2023; 27: 67 (https://doi.org/10.1186/s40824-023-00382-x)
- Michael Xavier Doss and Agapios Sachinidis, Current Challenges of iPSC-Based Disease Modeling and Therapeutic Implications. Cells 2019; 8: 403 (doi: 10.3390/cells8050403)
- Masahito Yoshihara et al., Genomic Instability of iPSCs: Challenges Towards Their Clinical Applications. Stem Cell Reviews 2016; 13: 7-16 (doi: 10.1007/s12015-016-9680-6)
- Corrine Ying Xuan Chua et al., Emerging immunomodulatory strategies for cell therapeutics. Trends in Biotechnology 2023; 41: 358-73 (https://doi.org/10.1016/j.tibtech.2022.11.008)
- Alexander Wiseman, Immunosuppressive Medications. Clinical Journal of the American Society of Nephrology 2015; 11: 332-43 (doi: 10.2215/CJN.08570814)
- Yash Sharma et al., The potential of immunomodulators in shaping the future of healthcare. Discover Medicine 2024; 1: 37 (https://doi.org/10.1007/s44337-024-00029-3)
- Margarita Bogomiakova et al., iPSC-derived cells lack immune tolerance to autologous NK-cells due to imbalance in ligands for activating and inhibitory NK-cell receptors. Stem Cell Research and Therapy 2023; 14: 77 (https://doi.org/10.1186/s13287-023-03308-5)
- Young Cha et al., Chapter 1 - Current reprogramming methods to generate high-quality iPSCs. Methods in iPSC Technology 2021; 9: 1-36 (https://doi.org/10.1016/B978-0-323-85766-6.00002-4)
- Jisu Park et al., The role of histone modifications: from neurodevelopment to neurodiseases. Signal Transduction and Targeted Therapy 2022; 7: 217 (https://doi.org/10.1038/s41392-022-01078-9)
- Haofei Wang et al., Direct cell reprogramming: approaches, mechanisms and progress. Nature Reviews Molecular Cell Biology 2021; 22: 410-24 (https://doi.org/10.1038/s41580-021-00335-z)
- Qian Zhang and Xuetao Cao, Epigenetic regulation of the innate immune response to infection. Nature Reviews Immunology 2019; 19: 417-32 (https://doi.org/10.1038/s41577-019-0151-6)
- Katarzyna Placek et al., Epigenetic reprogramming of immune cells in injury, repair, and resolution. The Journal of Clinical Investigation 2019; 129: 2994-3005 (doi: 10.1172/JCI124619)
- Mazhar Adli, The CRISPR toolkit for genome editing and beyond. Nature Communications 2018; 9: 1911 (https://doi.org/10.1038/s41467-018-04252-2)
- Jingyang Guan et al., Chemical reprogramming of human somatic cells to pluripotent stem cells. Nature 2022; 605: 325-31 (https://doi.org/10.1038/s41586-022-04593-5)
- Casey DuBose et al., Dynamic Features of Chromosomal Instability during Culture of Induced Pluripotent Stem Cells. Genes 2022; 13: 1157 (doi: 10.3390/genes13071157)
- Jose Braganca et al., Induced pluripotent stem cells, a giant leap for mankind therapeutic applications. World Journal of Stem Cells 2019; 11: 421-30 (doi: 10.4252/wjsc.v11.i7.421)
- Novalia Pishesha et al., A guide to antigen processing and presentation. Nature Reviews Immunology 2022; 22: 751-64 (https://doi.org/10.1038/s41577-022-00707-2)
- Tongbiao Zhao et al., Immunogenicity of induced pluripotent stem cells. Nature 2011; 474: 212-5 (doi: 10.1038/nature10135)
- Sarah Sun and Luis Barreiro, The epigenetic encoded memory of the innate immune system. Current Opinion in Immunology 2020; 65: 7-13 (doi: 10.1016/j.coi.2020.02.002)
- Stephanie Fanucchi et al., The Intersection of Epigenetics and Metabolism in Trained Immunity. Immunity 2021; 54: 32-43 (DOI: 10.1016/j.immuni.2020.10.011)
- Wendy Dong and Boris Kantor, Lentiviral Vectors for Delivery of Gene-Editing Systems Based on CRISPR/Cas: Current State and Perspectives. Viruses 2021; 13: 1288 (doi: 10.3390/v13071288)
- Ali Doga Yucel and Vadim Gladyshev, The long and winding road of reprogramming-induced rejuvenation. Nature Communications 2024; 15: 1941 (https://doi.org/10.1038/s41467-024-46020-5)
- Yohan Kim et al., Small-molecule-mediated reprogramming: a silver lining for regenerative medicine. Experimental & Molecular Medicine 2020; 52: 213-26 (https://doi.org/10.1038/s12276-020-0383-3)
- Jinlin Wang et al., Chemical reprogramming for cell fate manipulation: Methods, applications, and perspectives. Cell Stem Cell 2023; 30: 1130-47 (DOI: 10.1016/j.stem.2023.08.001)
- Masahito Yoshihara et al., Genomic Instability of iPSCs and Challenges in Their Clinical Applications. Advances in Experimental Medicine and Biology 2019; 1201: 23-47 (DOI: 10.1007/978-3-030-31206-0_2)
- Stephen Attwood and Michael Edel, iPS-Cell Technology and the Problem of Genetic Instability-Can It Ever Be Safe for Clinical Use? Journal of Clinical Medicine 2019; 8: 288 (doi: 10.3390/jcm8030288)
- Sean McCutcheon et al., Epigenome editing technologies for discovery and medicine. Nature Biotechnology 2024; 42: 1199-1217 (https://doi.org/10.1038/s41587-024-02320-1)
- Khaled Allemailem et al., Innovative Strategies of Reprogramming Immune System Cells by Targeting CRISPR/Cas9-Based Genome-Editing Tools: A New Era of Cancer Management. International Journal of Nanomedicine 2023; 18: 5531-59 (doi: 10.2147/IJN.S424872)
- Atilgan Yilmaz and Nissim Benvenisty, Defining Human Pluripotency. Cell Stem Cell 2019; 25: 9-22 (DOI: 10.1016/j.stem.2019.06.010)
- Tobias Deuse et al., Hypoimmunogenic derivatives of induced pluripotent stem cells evade immune rejection in fully immunocompetent allogeneic recipients. Nature Biotechnology 2019; 37: 252-58 (https://doi.org/10.1038/s41587-019-0016-3)
- Zhiqing Xu and Ming Chu, Advances in Immunosuppressive Agents Based on Signal Pathway. Frontiers in Pharmacology 2022; 13: 917162 (doi: 10.3389/fphar.2022.917162)
- Marek Wojtukiewicz et al., Inhibitors of immune checkpoints-PD-1, PD-L1, CTLA-4-new opportunities for cancer patients and a new challenge for internists and general practitioners. Cancer Metastasis Reviews 2021; 40: 949-82 (DOI: 10.1007/s10555-021-09976-0)
- Xing He and Chenqi Xu, Immune checkpoint signaling and cancer immunotherapy. Cell Research 2020; 30: 660-9 (https://doi.org/10.1038/s41422-020-0343-4)
- Tomokazu Sumida et al., The regulation and differentiation of regulatory T cells and their dysfunction in autoimmune diseases. Nature Reviews Immunology 2024; 24: 503-17 (https://doi.org/10.1038/s41577-024-00994-x)
- Huaigeng Xu et al., Targeted Disruption of HLA Genes via CRISPR-Cas9 Generates iPSCs with Enhanced Immune Compatibility. Cell Stem Cell 2019; 24: 566-78 (DOI: 10.1016/j.stem.2019.02.005)
- Alessia Gravina et al., Synthetic immune checkpoint engagers protect HLA-deficient iPSCs and derivatives from innate immune cell cytotoxicity. Cell Stem Cell 2023; 30: 1538-48 (DOI: 10.1016/j.stem.2023.10.003)
- Hanbing Wang et al., Beta2-microglobulin(B2M) in cancer immunotherapies: Biological function, resistance and remedy. Cancer Letters 2021; 517: 96-104 (https://doi.org/10.1016/j.canlet.2021.06.008)
- Julien Zuber and Megan Sykes, Mechanisms of Mixed Chimerism-Based Transplant Tolerance. Trends in Immunology 2017; 38: 829-43 (doi: 10.1016/j.it.2017.07.008)
- Cody Moorman et al., Emerging Therapeutics for Immune Tolerance: Tolerogenic Vaccines, T cell Therapy, and IL-2 Therapy. Frontiers in Immunology 2021; 12: 657768 (doi: 10.3389/fimmu.2021.657768)
- Mark Mannie et al., Tolerogenic vaccines: Targeting the antigenic and cytokine niches of FOXP3+ regulatory T cells. Cellular Immunology 2020; 355: 104173 (doi: 10.1016/j.cellimm.2020.104173)
- Liyang Lyu et al., Mapping the global landscape for induced pluripotent stem cells from patents and clinical trials. Nature Biotechnology 2024; 42: 563-9 (https://doi.org/10.1038/s41587-024-02196-1)
- Martinpacesa et al., Past, present, and future of CRISPR genome editing technologies. Cell 2024; 187: 1076-100 (DOI: 10.1016/j.cell.2024.01.042)
About The Author:
Jack (Jie) Huang, MD, Ph.D., is the chief scientist and founder/CEO at CSTEAM Biotechnology in Ohio, USA. He is also recognized as a medical science writer, an inventor, and a STEM educator. Huang completed his MD/Ph.D. at Shiga University of Medical Science (SUMS) in Japan in 2000. He then pursued postdoctoral training in immunology at the University of Tokyo, followed by a role as research assistant professor at Tohoku University. In the U.S., he served as a postdoctoral fellow at Oregon Health & Science University and as an oncologist and senior research scientist at Ohio State University Nationwide Children’s Hospital, focusing on oncology research until 2018, when he launched his company specializing in biological models and biochips. Additionally, Huang was vice president of the American Botanical Drug Association, director of the Stem Cell Engineering and Technology Research Center at the Industry Technology Research Institute of Chongqing University, and chief scientist of the International Institute of In Vitro Diagnostics at Chongqing Medical University. His research interests, which span genetic engineering biological models, gene-edited stem cells, immune cell drugs, and biochip technology, have led to numerous patents. He can be reached by email at csteam-biomed@hotmail.com.