How Predictive Can Regulatory Drug Abuse Potential Investigations Be in Animals?
By Anke Rosch, Boehringer Ingelheim
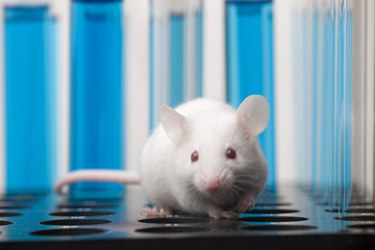
Is addiction attributed to “the animal or the human within us”1 or is substance abuse unique to humans? This question has intrigued me since the EMA guidance on Non-clinical Investigation Of The Dependence Potential Of Medicinal Products in 20062 and the U.S. FDA guidance for industry on the Assessment of Abuse Potential of Drugs in 20103 (draft) have requested the performance of dedicated studies in animals.
Regulatory Considerations
Both the EMA and FDA guidance made investigations mandatory on the abuse potential for CNS active drugs that are novel or that have a chemical structure and/or mechanism of action similar to known substances of abuse and for their major metabolites.2,3 Part 1 of this article series4 detailed early preclinical investigations enabling the assignment of drug candidates to this class of concern.
For preclinical abuse potential testing in animals, EMA2 defined first tier tests as primary and secondary pharmacodynamic (safety pharmacology) studies.5 These tests investigate CNS effects of a substance either related or unrelated to its therapeutic target, respectively, at therapeutic doses and above. As second tier tests, EMA2 identified preclinical in-vivo abuse potential studies. For simplicity, the FDA guidance3 summarized all preclinical in-vivo work under the term “animal abuse-related studies.”
First Tier Tests
As part of the core battery in safety pharmacology,5 the modified Irwin test6 or the functional observation battery7 in mice and rats is performed before Phase 1 clinical studies. They serve for general investigation of behavioral, neurological, and locomotor activity effects after drug treatment at therapeutic up to toxic doses. Effects are compared mostly to a vehicle but not to a positive control group, which is mandatory for preclinical abuse potential studies. These tests enable the separation of sedative or stimulant properties of a drug8 and the identification of abuse–related clinical signs such as head twitches or wet dog shakes.9 The results may support the choice of a positive control2, 3 for dedicated preclinical abuse potential studies, especially for drugs with a new mechanism of action (MoA).
Positive signals in preclinical locomotor activity investigations in rats and mice, such as decreases (depressant, e.g., morphine) or increases (stimulant, e.g., amphetamine), are a flag for further dedicated abuse potential studies. They need to be separated in their classification from anxiogenic,10 sedative, and anorectic effects or from a drug-induced disruption of working memory.11 Increased sensation-seeking in humans that preclinically translates to an increased spontaneous locomotor activity in a novel environment12 raises the vulnerability to addiction13 to substances such as cocaine14 and nicotine.15 Rats with a higher locomotor activity achieved higher levels of self-administration of, e.g., cocaine16 and amphetamine17 but not morphine.18 This inconsistency leaves the question open whether increased locomotor activity is predictive for a higher propensity to self-administer drugs19 or for differences in learning an operant task.20
Several publications have challenged the relevance of preclinical CNS findings for prediction of human adverse drug reactions. Olson and colleagues21 showed about 60% concordance based on 150 drugs in 2000, in contrast with Tamaki et al.22 with only 26% correlation based on 142 drugs in 2013. Meade et al. (2015)23 concluded that the rodent Irwin test/functional observation battery neither predicts nor detects most common adverse events in human Phase 1 (141 drugs).
How much of human addiction is shared with animals and can be modeled successfully?
Second Tier Tests
Literature presents addiction-like behavior in the wild, such as elephants enjoying the fermented fruits of the Marula tree in Africa,24 wallabies getting high on poppy plants,25 and big-horned sheep with preference for hallucinogenic lichen,26 thus supporting modeling in animals.
Dedicated tests requested by EMA2 and FDA,3 such as drug discrimination and intravenous self-administration (IVSA), are usually performed in non-standard rat strains after Phase 2 clinical studies. The selected dose range ideally results in a two- to three-fold multiple of the maximum human therapeutic plasma concentration (FDA)3 or is pushed up to the maximum tolerated dose (EMA)2 as long as the animal can successfully pass the test.
In the drug discrimination paradigm in a two-lever operant chamber, rats are trained with a known agent of abuse to discriminate “drug” (training drug-like) from “non-drug” (e.g., vehicle-like) by pressing one of two different levers. Various administration routes are feasible. The similarity between the new drug and the training drug is graded as full generalization (>80% drug appropriate lever pressing), partial generalization (60%-80%), or no generalization (<20 %).3
Predictive testing highly depends on the choice of a pharmacologically “similar” training drug.27,28 For example, rats trained with the µ-opioid agonist fentanyl showed generalization to other µ-opioid agonists but not to haloperidol, a dopamine D2 antagonist.29 Impressive test selectivity within a receptor system was proven with GABA modulating substances. Baboons trained with lorazepam showed generalization to other benzodiazepines, only partial generalization to barbiturates, and no generalization to the minor tranquillizer meprobamate.30, 31, 32 This raises doubts whether we can prove generalization at all when a drug with a new MoA shows only a marginal neuropharmacological overlap with the training drug and raises the question how we should interpret partial generalization.
The training and positive control drugs for compounds with a new MoA are often selected in relation to their target or off-target activity, or animals are tried to be trained by the drug candidate. Ator and Griffith33 characterized the use of a training drug and positive control based on the similarity of effects of the drug candidate on general condition in animals like sedation or stimulation as subordinate.
Horton and colleagues34 showed in a literature search an overall concordance of 69% between the drug discrimination results and the Controlled Substances Act (CSA)35 scheduling status based on 100 drugs.
The investigation of rewarding properties of new drugs, e.g., by the intravenous self-administration paradigm, is less dependent on the choice of the training drug.
The self-administration paradigm as a form of an operant conditioning investigates whether a new drug produces a similar pleasurable psychoactive experience, like a known drug of abuse. After training with food rewards and a reinforcing scheduled training drug, rats equipped with chronic indwelling jugular cannula may repeatedly start self-administration of the compound by lever pressing.
Because of the frequent failure of drugs developed for substance use disorder,36 this test scenario was caught in the crossfire, starting with the “Rat Park Project”37 in 1970. A series of studies38,39 challenged whether the more frequent decision of isolated rats to self-administer morphine compared to socially housed rats indicated the rewarding properties of the drug or a lack of choice, now known to be shared with human drug addicts.40,41 Investigations by Ahmed and colleagues supported the conclusion that we cannot model human addiction, showing that most rats (85%-90%) stopped their escalating drug intake when they had free access to an alternative reinforcer such as saccharin.42,43 Based on historical evaluations of individuals over five years, a small minority of 8.7% of rats44 expressed a continuous preference for intravenous cocaine. The number of human cocaine users resulting in less than 10% becoming addicted45 shows surprising similarities with the situation in animals.
There is proof, however, that nearly all drugs abused in humans could be self-administered46, 47 in animals. O’Connor and colleagues showed in literature concordant findings in the rat self-administration study with at least one clinical abuse indicator in 64 (90.1%) of 71 drugs.48 Fixed ratio or progressive ratio IVSA studies could predict the later scheduling status in 78% or 71% of cases, respectively.34 In contrast, a poster presented by FDA representatives in 201949 reviewed the outcome of preclinical abuse potential studies between 2009 and 2019 for eight molecular entities with a new MoA supervised in the CSA.35 Five out of six drugs tested in the IVSA paradigm and four out of eight monitored in the drug discrimination assay in rats and/or monkeys showed negative results in contrast to clinical studies.
Conclusions
The article describes a selection of animal models for preclinical abuse potential testing that are mentioned in EMA2 and FDA3 guidelines.
Numerous publications have challenged the validity of preclinical models for the development of new medications. This does not surprise. The multifaceted nature of this disorder in humans as a neurochemical brain disease and a disorder of choice affected by environmental factors50 is hard to model in animals.
For preclinical abuse potential testing, the predictivity is considered acceptable based on retrospectively generated, concordant results for substances known to be addictive. When a preclinical decision about the abuse potential of a compound needs to be done prospectively, the lack of appropriate positive control and training substances, equivocal findings such as partial generalization, and the variability among the study animals may aggravate classification. Extended testing with more than the standard preclinical models, including more than one training /positive control drug for substances with a new MoA, collides with the goals of speeding up the development of new medications and of reducing the number of animals.
Horton and colleagues34 dampened the enthusiastic hope for unequivocal results of human abuse potential studies. They showed that combinations of, e.g., preclinical with clinical studies have a similar predictivity for the scheduling status as one of the individual studies alone. I am excited to see further progress of these testing strategies within the next decade in relation to the conceptualization of substance use disorder as unique to humans or successfully testable in animal models.
References
- Ahmed S.H.‚ A walk on the wild side of addiction: the history and significance of animal models. In: Pickard H., Ahmed S.H., editors. Routledge Handbook on Philosophy and Science of Addiction. London and New York: Routledge; 2018; 192-203.
- EMEA/CHMP, European Medicine Agency, Committee for Medicinal Products for Human Use, 2006. Guidance on the non-clinical Investigation of the Dependence Potential of Medicinal Products (https://www.ema.europa.eu/docs/en_GB/document_library/Scientific_guideline/2009/09/WC
500003360.pdf, (accessed 26 April 2023). - FDA/CDER, U.S. Department of Health and Human Services, Food and Drug Administration, Center for Drug Evaluation and Research, 2010. Guidance for Industry on the Assessment of Abuse Potential of Drugs https://www.fda.gov/downloads/drugs/guidancecomplianceregulatoryinformation/guidances/
ucm198650.pdf, (Accessed 26 April 2023). - Search for Similarity: Regulatory Considerations Of Drug Abuse Potential Investigations, Clinical Leader, 14 Oct 2022, Search For Similarity Regulatory Considerations Of Drug Abuse Potential Investigations (clinicalleader.com) (accessed 26 April 2023)
- S7A: Safety pharmacological studies for human pharmaceuticals, CPMP/ICH/539/00, GUIDELINE FOR GOOD CLINICAL PRACTICE (ich.org) (2001) (accessed on 26 April 2023)
- Irwin S. Comprehensive observational assessment: Ia. A systematic, quantitative procedure for assessing the behavioral and physiologic state of the mouse. Psychopharmacologia. 1968 Sep 20;13(3):222-57. doi: 10.1007/BF00401402. PMID: 5679627.
- Mattsson JL, Spencer PJ, Albee RR. A Performance Standard for Clinical and Functional Observational Battery Examinations of Rats. Journal of the American College of Toxicology. 1996;15(3):239-254. doi:10.3109/10915819609008716.
- Heal DJ, Gosden J, Smith SL. Evaluating the abuse potential of psychedelic drugs as part of the safety pharmacology assessment for medical use in humans. Neuropharmacology. 2018 Nov;142:89-115. doi: 10.1016/j.neuropharm.2018.01.049. Epub 2018 Feb 8. PMID: 29427652.
- Halberstadt AL, Geyer MA. Characterization of the head-twitch response induced by hallucinogens in mice: detection of the behavior based on the dynamics of head movement. Psychopharmacology (Berl). 2013 Jun;227(4):727-39. doi: 10.1007/s00213-013-3006-z. Epub 2013 Feb 14. PMID: 23407781; PMCID: PMC3866102.
- Gentsch C, Lichtsteiner M, Feer H. Open field and elevated plus-maze: a behavioural comparison between spontaneously hypertensive (SHR) and Wistar-Kyoto (WKY) rats and the effects of chlordiazepoxide. Behav Brain Res. 1987 Aug;25(2):101-7. doi: 10.1016/0166-4328(87)90003-9. PMID: 3675823.
- Jeltsch H, Bertrand F, Lazarus C, Cassel JC. Cognitive performances and locomotor activity following dentate granule cell damage in rats: role of lesion extent and type of memory tested. Neurobiol Learn Mem. 2001 Jul;76(1):81-105. doi: 10.1006/nlme.2000.3986. PMID: 11525255.
- Blanchard MM, Mendelsohn D, Stamp JA. The HR/LR model: Further evidence as an animal model of sensation seeking. Neurosci Biobehav Rev. 2009 Jul;33(7):1145-54. doi: 10.1016/j.neubiorev.2009.05.009. Epub 2009 Jun 2. PMID: 19497336.
- Zuckerman, M. (2007). Sensation Seeking and Substance Use and Abuse: Smoking, Drinking, and Drugs. In M. Zuckerman, Sensation seeking and risky behavior (pp. 107–143). American Psychological Association, https://doi.org/10.1037/11555-004
- O'Connor SL, Aston-Jones G, James MH. The sensation seeking trait confers a dormant susceptibility to addiction that is revealed by intermittent cocaine self-administration in rats. Neuropharmacology. 2021 Sep 1;195:108566. doi: 10.1016/j.neuropharm.2021.108566. Epub 2021 Apr 20. PMID: 33862028; PMCID: PMC8410649.
- Martin CA, Kelly TH, Rayens MK, Brogli BR, Brenzel A, Smith WJ, Omar HA. Sensation seeking, puberty, and nicotine, alcohol, and marijuana use in adolescence. J Am Acad Child Adolesc Psychiatry. 2002 Dec;41(12):1495-502. doi: 10.1097/00004583-200212000-00022. PMID: 12447037.
- Belin D, Berson N, Balado E, Piazza PV, Deroche-Gamonet V. High-novelty-preference rats are predisposed to compulsive cocaine self-administration. Neuropsychopharmacology. 2011 Feb;36(3):569-79. doi: 10.1038/npp.2010.188. Epub 2010 Oct 27. PMID: 20980989; PMCID: PMC3055686.
- Piazza PV, Deminière JM, Maccari S, Mormède P, Le Moal M, Simon H. Individual reactivity to novelty predicts probability of amphetamine self-administration. Behav Pharmacol. 1990;1(4):339-345. doi: 10.1097/00008877-199000140-00007. PMID: 11175418.
- Swain Y, Muelken P, LeSage MG, Gewirtz JC, Harris AC. Locomotor activity does not predict individual differences in morphine self-administration in rats. Pharmacol Biochem Behav. 2018 Mar;166:48-56. doi: 10.1016/j.pbb.2018.01.008. Epub 2018 Feb 2. PMID: 29409807; PMCID: PMC5821250.
- Piazza PV, Deminière JM, Le Moal M, Simon H. Factors that predict individual vulnerability to amphetamine self-administration. Science. 1989 Sep 29;245(4925):1511-3. doi: 10.1126/science.2781295. PMID: 2781295.
- Mitchell JM, Cunningham CL, Mark GP. Locomotor activity predicts acquisition of self-administration behavior but not cocaine intake. Behav Neurosci. 2005 Apr;119(2):464-72. doi: 10.1037/0735-7044.119.2.464. PMID: 15839792; PMCID: PMC4327862.
- Olson H, Betton G, Robinson D, Thomas K, Monro A, Kolaja G, Lilly P, Sanders J, Sipes G, Bracken W, Dorato M, Van Deun K, Smith P, Berger B, Heller A. Concordance of the toxicity of pharmaceuticals in humans and in animals. Regul Toxicol Pharmacol. 2000 Aug;32(1):56-67. doi: 10.1006/rtph.2000.1399. PMID: 11029269.
- Tamaki C, Nagayama T, Hashiba M, Fujiyoshi M, Hizue M, Kodaira H, Nishida M, Suzuki K, Takashima Y, Ogino Y, Yasugi D, Yoneta Y, Hisada S, Ohkura T, Nakamura K. Potentials and limitations of nonclinical safety assessment for predicting clinical adverse drug reactions: correlation analysis of 142 approved drugs in Japan. J Toxicol Sci. 2013;38(4):581-98. doi: 10.2131/jts.38.581. PMID: 23824014.
- Mead AN, Amouzadeh HR, Chapman K, Ewart L, Giarola A, Jackson SJ, Jarvis P, Jordaan P, Redfern W, Traebert M, Valentin JP, Vargas HM. Assessing the predictive value of the rodent neurofunctional assessment for commonly reported adverse events in phase I clinical trials. Regul Toxicol Pharmacol. 2016 Oct;80:348-57. doi: 10.1016/j.yrtph.2016.05.002. Epub 2016 May 4. PMID: 27155597.
- Siegel, R.K., Brodie, M. Alcohol self-administration by elephants. Bull. Psychon. Soc. 22, 49–52 (1984), https://doi.org/10.3758/BF03333758.
- Briant B, Knights K, Miners, JO. Pharmacology for Health professionals, 4th edition, 2015, 9780729541701 (paperback) Mosby , Elsevier Australia. Page 453.
- Haynes A. The animal world has its junkies too. Pharm J 2010;285:723-724.
- McMahon LR. The rise (and fall?) of drug discrimination research. Drug Alcohol Depend. 2015 Jun 1;151:284-8. doi: 10.1016/j.drugalcdep.2015.04.001. PMID: 26207268; PMCID: PMC4879885.
- Lynch WJ, Nicholson KL, Dance ME, Morgan RW, Foley PL. Animal models of substance abuse and addiction: implications for science, animal welfare, and society. Comp Med. 2010 Jun;60(3):177-88. PMID: 20579432; PMCID: PMC2890392.
- Colpaert FC, Lal H, Niemegeers CJ, Janssen PA. Investigations on drug produced and subjectively experienced discriminative stimuli. I. The fentanyl cue, a tool to investigate subjectively experience narcotic drug actions. Life Sci. 1975 Mar 1;16(5):705-15. doi: 10.1016/0024-3205(75)90347-1. PMID: 1123967.
- Ator, N.A., Griffiths, R.R., 1989. Asymmetrical cross-generalizationin drug discrimination with lorazepam and pentobarbital training conditions. Drug Dev. Res. 16, 355-364.
- Ator NA, Griffiths RR. Differential generalization to pentobarbital in rats trained to discriminate lorazepam, chlordiazepoxide, diazepam, or triazolam. Psychopharmacology (Berl). 1989;98(1):20-30. doi: 10.1007/BF00442001. PMID: 2567033.
- Ator NA, Griffiths RR. Selectivity in the generalization profile in baboons trained to discriminate lorazepam: benzodiazepines, barbiturates and other sedative/anxiolytics. J Pharmacol Exp Ther. 1997 Sep;282(3):1442-57. PMID: 9316858.
- Ator NA, Griffiths RR. Principles of drug abuse liability assessment in laboratory animals. Drug Alcohol Depend. 2003 Jun 5;70(3 Suppl):S55-72. doi: 10.1016/s0376-8716(03)00099-1. PMID: 12759197.
- Horton DB, Potter DM, Mead AN. A translational pharmacology approach to understanding the predictive value of abuse potential assessments. Behav Pharmacol. 2013 Sep;24(5-6):410-36. doi: 10.1097/FBP.0b013e3283644d2e. PMID: 23873088.
- U.S. Department of Justice, Drug Enforcement Administration, Diversion Control Division, Drug & Chemical Evaluation section. February 2023. List of scheduling actions, controlled substances, regulated chemicals. Controlled Substances - Alpha Order (usdoj.gov) (accessed 26 April 2023)
- Heilig M, Epstein DH, Nader MA, Shaham Y. Time to connect: bringing social context into addiction neuroscience. Nat Rev Neurosci. 2016 Sep;17(9):592-9. doi: 10.1038/nrn.2016.67. Epub 2016 Jun 9. PMID: 27277868; PMCID: PMC5523661.
- Alexander BK, Coambs RB, Hadaway PF. The effect of housing and gender on morphine self-administration in rats. Psychopharmacology (Berl). 1978 Jul 6;58(2):175-9. doi: 10.1007/BF00426903. PMID: 98787.
- Hadaway PF, Alexander BK, Coambs RB, Beyerstein B. The effect of housing and gender on preference for morphine-sucrose solutions in rats. Psychopharmacology (Berl). 1979;66(1):87-91. doi: 10.1007/BF00431995. PMID: 120547.
- Alexander BK, Beyerstein BL, Hadaway PF, Coambs RB. Effect of early and later colony housing on oral ingestion of morphine in rats. Pharmacol Biochem Behav. 1981 Oct;15(4):571-6. doi: 10.1016/0091-3057(81)90211-2. PMID: 7291261.
- Freed CR. In the Spirit of Selden Bacon: The Sociology Of Drinking and Drug Problems. Sociol Compass. 2010 Oct;4(10):856-868. doi: 10.1111/j.1751-9020.2010.00325.x. Epub 2010 Oct 3. PMID: 30555527; PMCID: PMC6291215.
- Ahmed SH, Badiani A, Miczek KA, Müller CP. Non-pharmacological factors that determine drug use and addiction. Neurosci Biobehav Rev. 2020 Mar;110:3-27. doi: 10.1016/j.neubiorev.2018.08.015. Epub 2018 Sep 1. PMID: 30179633; PMCID: PMC6395570.
- Ahmed SH, Badiani A, Miczek KA, Müller CP. Non-pharmacological factors that determine drug use and addiction. Neurosci Biobehav Rev. 2020 Mar;110:3-27. doi: 10.1016/j.neubiorev.2018.08.015. Epub 2018 Sep 1. PMID: 30179633; PMCID: PMC6395570.
- Ahmed SH. Individual decision-making in the causal pathway to addiction: contributions and limitations of rodent models. Pharmacol Biochem Behav. 2018 Jan;164:22-31. doi: 10.1016/j.pbb.2017.07.005. Epub 2017 Jul 12. PMID: 28709784.
- Ahmed SH. Validation crisis in animal models of drug addiction: beyond non-disordered drug use toward drug addiction. Neurosci Biobehav Rev. 2010 Nov;35(2):172-84. doi: 10.1016/j.neubiorev.2010.04.005. Epub 2010 Apr 22. PMID: 20417231.
- Reboussin BA, Anthony JC. Is there epidemiological evidence to support the idea that a cocaine dependence syndrome emerges soon after onset of cocaine use? Neuropsychopharmacology. 2006 Sep;31(9):2055-64. doi: 10.1038/sj.npp.1301037. Epub 2006 Feb 8. PMID: 16482089; PMCID: PMC2575802.
- Stitzer M. Classic texts revisited. Re: Similarities in Animal and Human Drug-Taking Behavior by Roland R. Griffiths, George E. Bigelow, Jack E. Henningfield, in: Advances in Substance Abuse, Volume 1, JAI Press Inc., 1980, pp. 190. Addiction. 2005 Aug;100(8):1196-7. doi: 10.1111/j.1360-0443.2005.01237.x. PMID: 16042651.
- van Ree JM, Slangen JL, de Wied D. Intravenous self-administration of drugs in rats. J Pharmacol Exp Ther. 1978 Mar;204(3):547-57. PMID: 633066.
- O'Connor EC, Chapman K, Butler P, Mead AN. The predictive validity of the rat self-administration model for abuse liability. Neurosci Biobehav Rev. 2011 Jan;35(3):912-38. doi: 10.1016/j.neubiorev.2010.10.012. Epub 2010 Oct 29. PMID: 21036191.
- Hawkins G, Calderon S, Chiapperino D. Are animal studies sufficient to assess the abuse potential of a new molecular entity? Journal of Pharmacological and Toxicological Methods, Volume 105, September 2020, 106703, https://doi.org/10.1016/j.vascn.2020.106703.
- Vandaele Y, Daeppen JB. From concepts to treatment: a dialog between a preclinical researcher and a clinician in addiction medicine. Transl Psychiatry. 2022 Sep 21;12(1):401. doi: 10.1038/s41398-022-02177-5. PMID: 36130939; PMCID: PMC9492712.
About The Author:
Anke Rosch is a board-certified pharmacologist and toxicologist working at Boehringer Ingelheim Pharma GmbH & Co. KG. A doctor of veterinary medicine, she has more than 20 years of experience in the pharmaceutical industry and has special expertise in safety pharmacology. Anke can be reached at ankerosch.PharmacolTox@t-online.de.