How Can Nanomedicine Innovations Combat Cancer?
By Tim Sandle, Ph.D.
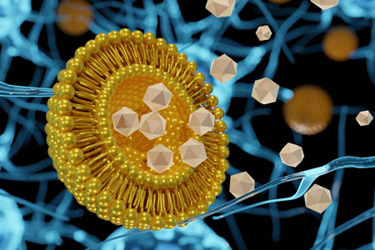
For the past two decades, nanotechnology has promised a revolution in medicine, especially in creating “smart” ways to deliver medicines to specific targets for more effective treatments. Scientists are working on various types of nanoparticles, especially to diagnose and treat cancer. This article considers some of the key innovations within the field during the past year and what future developments are promising.
What Is Nanomedicine?
Nanomedicine refers to any medicine that includes a component of artificially constructed nanotechnology (“artificial” differentiates the science from bacteria and viruses). Nanotechnology is a size-based concept describing technologies measured below 1,000 nanometers (one nanometer is one-millionth of a millimeter). The application is based on the empirical advantages of nanoparticles, which can be manipulated to improve the behavior of drug substances.
These advantages are manifested through improved drug delivery. This overcomes three obstacles met by conventional medicine:
- Several types of drugs have little solubility in water and the human body struggles to absorb enough to treat the condition.
- While some drug molecules are absorbed well, often the body removes the drug before it has had long enough to provide a benefit or side-effects are more likely to develop.
- Drugs often miss their intended target, or the drug is delivered to the intended target and healthy cells, causing unintended damage to other parts of the body.
Nanotechnology overcomes absorption issues due to the size of the medicine and because nanoparticles can hold drugs that would otherwise become insoluble or quickly degrade in the bloodstream tightly on the surface of the particle. In addition, due to their small size, the nanoparticles can overcome biological barriers such as membranes, skin, and the small intestine, which would usually prevent the drug from reaching its target.1
In terms of delivering the medicine for the required timeframe, using a nano-engineered drug carrier enables the slow release of the active ingredient. Nanotechnology also enables medicines to be delivered with greater precision, with the drug targeted specifically to the areas of the body that need treatment.
Nanoparticles of an active ingredient are typically covered by a biomolecular multilayer (such as a protein corona). This outer layer alters the physiochemical properties, pharmacokinetics, and toxicity profile of the nanoparticles, enabling targeted delivery and increased survival time within the body.2
The Chicken Or The Egg: Do We Need Nanostructure Innovation Or Suitable Drug Candidates First?
Despite the advantages outlined above, nanomedicine has not been successful in cancer treatment. This is largely due to poor clinical translation from preclinical models to cancer patients.3 To overcome this, nanoparticles need to be strengthened further to protect the paths that nanomedicines need to take within the human body.
Scientists from Wyss Institute, Harvard, have created more stable nanostructures that can assemble biomolecules with different functions. This involves developing DNA nanostructures that can bind and assemble biomolecules into multifunctional structures. The specifically developed “DNA origami” is programmed to assemble into rigid square-lattice blocks. One such DNA nanostructure has been fashioned to create a branched structure with four ends. Researchers have succeeded in binding and collecting different antibodies that can guide the body’s T cells to attack cancer cells more intensively.4 The DNA nanostructure is made sufficiently stable by coating the DNA with a small molecule unobtrusive neutralizing agent called PEG-oligolysine. This positively charged chemical covers multiple negative DNA charges at once to create a stable “electrostatic net.” Stability is further enhanced by applying glutaraldehyde as a chemical cross-linking reagent.
An alternative to DNA is artificial oligonucleotides, which can be modified to form the nanostructure. An example comes from Aarhus University, using acyclic L-threoninol nucleic acid (aTNA). This is created when a naturally occurring sugar molecule (deoxyribose) is replaced with an artificial sugar molecule (acyclic L-threoninol). This strengthens the overall structure and minimizes the potential for degradation of the molecule in the blood. A further advantage is that these oligonucleotides do not elicit an immune response. Trials are taking place using the artificial oligonucleotides with a biomolecule with a high specificity to breast cancer cells.5
Improving the robustness of nanostructures remains academic unless new drug candidates are developed. This research area is gathering steam, including at the University of Arkansas, which has developed a drug candidate that kills triple negative breast cancer cells (an aggressive form of cancer that cannot be treated with receptor-targeted therapy). This involves a co-formation composed of a relatively new class of nanomaterials, termed metal-organic frameworks, with a photodynamic therapy drug. The resultant compound is a nano-porous material that targets and kills tumor cells.6
Photodynamic therapy uses a photosensitizer that, upon irradiation by light, generates toxic reactive oxygen species that kill cancer cells. The critical step is bio-conjugating the nanomaterials with the ligands (binding molecules) of the drug.
FDA’s New Guidance Will Help Move The Field Forward
As with any new medical innovation, a regulatory framework is required, and the FDA has recently issued guidance7 for the development of nanotechnology for use as active ingredients or inactive ingredients, including carriers loaded with an active ingredient. The guidance requires a risk-based approach to be adopted to drive safety and efficacy, as well as setting out the requirements for each drug application. There are three important risk assessment areas:
- Product stability: Here, the developer is advised to identify the potential factors that could impact the product performance, including interactions of nanomaterial properties. This requires scientific evaluation of the physical and chemical changes in the material during handling and storage.
- Safety: Often, the nanomaterial’s safety is not able to be fully demonstrated by existing safety data with respect. Therefore, an additional assessment is required relating to the level of exposure, duration of exposure, and route of administration.
- Efficacy: Especially for nanomaterials formed of complex structures involving multiple components or compartments, ligands, and coatings.
Nanoparticles-based drug delivery systems show promising therapeutic efficacy in cancer. To increase targetability to tumors, nanoparticles need to be sufficiently robust to survive the human body, to reach the correct target, and to be functionalized with a suitable drug. To ensure specificity and to safeguard patients against any negative influence from the delivery mechanism, a strong regulatory framework is timely and necessary.
References
- Ma, W., Saccardo, A., Roccatano, D., Aboagye-Mensah, D. et al Modular assembly of proteins on nanoparticles. Nature Communications, 2018; 9 (1) DOI: 10.1038/s41467-018-03931-4
- Berger, S., Berger, M., Bantz, C., Maskos, M., Wagner, E. Performance of nanoparticles for biomedical applications: The in vitro/in vivo discrepancy. Biophysics Reviews, 2022; 3 (1): 011303 DOI: 10.1063/5.0073494
- Song, S., Bugada, L., Li, R. et al Albumin nanoparticle containing a PI3Kγ inhibitor and paclitaxel combined with α-PD1 induces tumor remission of breast cancer in mice. Science Translational Medicine, 2022; 14 (643) DOI: 10.1126/scitranslmed.abl3649
- Anastassacos, F., Zhao, Z., Zeng, Y., Shih. W. Glutaraldehyde Cross-Linking of Oligolysines Coating DNA Origami Greatly Reduces Susceptibility to Nuclease Degradation, J. Am. Chem. Soc. 2020, 142, 7, 3311–3315
- Märcher, A., Kumar, V., Andersen, V. et al. Functionalized Acyclic ( l )‐Threoninol Nucleic Acid Four‐Way Junction with High Stability In Vitro and In Vivo. Angewandte Chemie International Edition, 2022; DOI: 10.1002/anie.202115275
- Sakamaki, Y., Ozdemir, J., Perez, A. et al Maltotriose Conjugated Metal–Organic Frameworks for Selective Targeting and Photodynamic Therapy of Triple Negative Breast Cancer Cells and Tumor Associated Macrophages. Advanced Therapeutics, 2020; 2000029 DOI: 10.1002/adtp.202000029
- FDA. Drug Products, Including Biological Products, that Contain Nanomaterials Guidance for Industry, U.S. Department of Health and Human Services, April 2022: https://www.fda.gov/media/157812/download
About The Author:
Tim Sandle, Ph.D., is a pharmaceutical professional with wide experience in microbiology and quality assurance. He is the author of more than 30 books relating to pharmaceuticals, healthcare, and life sciences, as well as over 170 peer-reviewed papers and some 500 technical articles. Sandle has presented at over 200 events and he currently works at Bio Products Laboratory Ltd. (BPL), and he is a visiting professor at the University of Manchester and University College London, as well as a consultant to the pharmaceutical industry. Visit his microbiology website at https://www.pharmamicroresources.com.