How Can 3D Bioprinting Advance Drug Discovery?
By Tim Sandle, Ph.D.
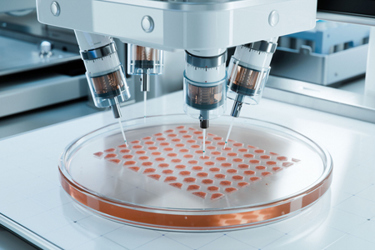
Biological printing (bioprinting) is a form of additive manufacturing that utilizes living cells, proteins, and nutrients as the basis of raw materials for manufacturing biomaterials for medicine and samples for the use in pharmaceutical development. A future goal is to use it to create entire organs for transplants, since 3D bioprinted models can capture human physiological responses better than two-dimensional cultures and animal models. In the meantime, the pharmaceutical sector has been taking advantage of this technology.
For pharmaceutical compound bioprinting, the process connects innovations in 3D printing with pharmaceutical sciences. The primary driver is the potential for furthering precision (personalized) medicines, as well as aiding drug development and creating customized drug delivery systems.
While 3D bioprinting remains relatively novel, further advances have taken technology toward 4D — the inclusion of time.
What Is 3D Bioprinting?
Three-dimensional printing (or additive manufacturing) is now a relatively established method of fabrication. Bioprinting (sometimes called “biofabrication”) is based on three manufacturing principles: subtractive (lithography), formative (drying in wells), and additive manufacturing.1
The general process enables the production of 3D structures based on custom-made models designed with computer-aided design software. Typically associated with plastic items for industry or general consumer use, the technology has made inroads into pharmaceuticals, for both designing medical devices and rendering three-dimensional biological structures. This involves the processing of living cells, growth factors, or other biological substances.2
Bioinks
Bioinks are the basis of the materials used to produce engineered 3D printed biomaterials. These inks are composed of the cells that are being used, sometimes in tandem with materials that envelope the cells (such as biopolymer gels). Bioinks have shear-thinning and rapid self-healing properties that enable smooth extrusion-based printing with high resolution and high fidelity. The printed bioconstructs, after further stabilization by light-based crosslinking, remain intact while, for example, bending, twisting or undergoing any number of multiple deformations.
Lund University recently advanced the use of bioinks, designing a material that allows small human-sized airways to be 3D-bioprinted. These were based on patient cells, intended to be biocompatible and to support new blood vessel growth.3
3D Success In Drug Testing
Certain drug substances may benefit from testing using 3D bioprinted materials. There are several advantages, including the ability of the process to render the flexible spatiotemporal deposition of cells, which assists with accurate bioprinting of tissues, each possessing a defined geometry and architecture. Coupling the fabrication process with digital technology, such as medical imaging data, aids the structural accuracy of the manufactured material.4
Further advances in the technology enable the creation of tissue models formed of multi-cell-laden materials (such as bioinks incorporated with multiple cells relevant to an organ). This helps to produce not only a functioning replication of human physiology but also disease pathophysiology.5 This leads to the development of biomimetic microenvironments capable of simulating cellular cross-talks, so pharmaceutical researchers can assess pathogenesis and pathological processes to determine where novel therapeutic targets can be discovered or directed.6
3D Success In Preclinical Studies And Clinical Trials
While developments remain at an early stage, a paper by Yang and colleagues suggests that some lead identification and preclinical studies will benefit from 3D bioprinting.7 This is because 3D bioprinted models can be utilized in high-throughput screening (HTS) systems. In addition, such models can generate efficacy and safety data that closely resemble clinical observations. The authors report there are several clinical trials in cancer therapies seeking to evaluate the predictive ability of 3D bioprinted models by comparing human and model responses to the same chemotherapy.
As part of such developments, researchers at Penn State 3D bioprinted breast cancer tumors and treated them in a study to better understand the disease that is one of the leading causes of mortality worldwide.8 The Penn State method used a technique called aspiration-assisted bioprinting to precisely locate tumors in three dimensions and create the tissue. The researchers then formed the tissue into a multi-scale vascularized breast tumor model with blood vessels, which they discovered responded to chemotherapy and cell-based immunotherapeutics. The accuracy of the tumor model was assessed by treating it with doxorubicin, an anthracycline-based chemotherapeutic drug commonly used for treating breast cancer. The reactions were deemed satisfactory, paving the way to explore new drug therapies.9
3D Success In The 4th Dimension
These important advances can be improved by accounting for time. To understand how a candidate drug works and how biological material reacts, we have to understand time. To paraphrase Einstein: to know where you are, you have to know what time it is. In the context of this article, to completely describe an event as a biological reaction, we need to know more than just where it occurs; we also need to know when it occurs.
The fourth dimension — shape transformation over time — can be incorporated into 3D models by incorporating materials that enable printed constructs to morph multiple times in a preprogrammed or on-demand manner in response to external signals.10 This concept extends to 3D biological structures that can transform their shapes or behavior under various stimuli.11 These include temperature, swelling behavior, pH, Ca2+ concentration, humidity, electric fields, light, and magnetic fields.
An example of how 4D printing can aid pharmaceutical research is tissue engineering, such as creating biomimetic blood vessels. To achieve these constructs, different cell types, such as endothelial cells, fibroblasts, and mesenchymal stem cells, can be combined with hydrogels and 4D printed to create tubular structures that mimic the changing properties of vasculature.12 This construct allows blood pressure medications to be developed, tracking how medications can treat these conditions in real time, to cite just one example.
A second example is creating materials capable of mimicking complex tumor environments to create in vitro dynamic models for the study of cancers.13 In one experiment, scientists fabricated curved hydrogel microstructures, made of photopatterned bilayers of poly (ethylene glycol) diacrylate (PEGDA). These possessed cell-stimulatory properties and enabled researchers to study the material reaction, over time, to sensitive peptide sequences.
A third area is drug release: optimizing medicines that can release drugs at the most suitable time point as well as in the optimal anatomical location. This requires control over both spatial and temporal delivery. Four-dimensional bioprinting technologies offer the means to streamline the development process.11 Here, 4D bioprinting can enable the precise control of the spatial dissemination of different delivery mechanisms, including systems capable of self-folding to encapsulate and release drugs or cells in a programmable manner (including an alginate-based hydrogel, sensitive to light and able to fold into complex shapes depending on the light exposure induced after printing).14
Other nanostructures can be developed to respond to micro-environmental changes. including osmolarity, temperature, humidity, light, and electric and magnetic stimuli. As an example, ETH Zürich engineered nano-hydrogels combined with non-cytotoxic photo-cross-linkable methacrylated gelatin (GelMA). Designed for targeted drug delivery, the nano-hydrogels were designed to be magnetically responsive through their surface being infused with magnetic nanoparticles.15
Other 4D printing applications include the fabrication of cardiac microtissues, shape-memory tracheal-bronchial stents, and cell-laden hydrogels for 3D human cancer spheroids. These present additional future-state opportunities for an improved understanding of disease and new treatment regimens or curative medications.
References
- Ashammakhi N, Ahadian S, Zengjie F, et al. Advances and future perspectives in 4D bioprinting. Biotechnol J. 2018;13(12):e1800148
- Pramanick, A., Hayes, T. Sergis, V. et al. 4D Bioprinting Shape‐Morphing Tissues in Granular Support Hydrogels: Sculpting Structure and Guiding Maturation. Advanced Functional Materials, 2024; DOI: 10.1002/adfm.202414559
- De Santis, M., Alsafadi, H., Tas, S. et al. Extracellular‐Matrix‐Reinforced Bioinks for 3D Bioprinting Human Tissue. Advanced Materials, 2020; 33 (3): 2005476 DOI: 10.1002/adma.202005476
- Peng, W., Datta, P., Ayan, B. et al. 3D bioprinting for drug discovery and development in pharmaceutics, Acta Biomater., 57 (2017), pp. 26-46
- Parihar, A. Pandita, V., Khan, 3D printed human organoids: high throughput system for drug screening and testing in current COVID-19 pandemic, Biotechnol. Bioeng., 119 (2022): 2669-2688
- Yi, H., Kim, H., Kwon, J. Application of 3D bioprinting in the prevention and the therapy for human diseases, Signal Transduct. Target., 6 (2021): 177
- Yang, K., Wang, L., Vijayavenkataraman, S. Recent applications of three-dimensional bioprinting in drug discovery and development, Advanced Drug Delivery Reviews, 2024; 214: 115456,
- Neufeld, L., Yeini, E., Reisman, N. Microengineered perfusable 3D-bioprinted glioblastoma model for in vivo mimicry of tumor microenvironment. Science Advances, 2021; 7 (34): eabi9119
- Dey, M., Kim, M., Dogan, M. et al. Chemotherapeutics and CAR‐T Cell‐Based Immunotherapeutics Screening on a 3D Bioprinted Vascularized Breast Tumor Model. Advanced Functional Materials, 2022; 2203966
- Ding, A., Jeon, O., Cleveland, D. et al. Jammed Micro‐Flake Hydrogel for Four‐Dimensional Living Cell Bioprinting. Advanced Materials, 2022; 2109394
- Li, Y-C., Zhang, Y., Akpek, A. et al. 4D bioprinting: the next-generation technology for biofabrication enabled by stimuli-responsive materials, Biofabrication, 9 (1): DOI 10.1088/1758-5090/9/1/012001
- Miri AK, Mirzaee I, Hassan S, et al. Effective bioprinting resolution in tissue model fabrication. Lab Chip. 2019;19(11):2019–2037
- Kwag HR, Serbo JV, Korangath P, et al. A self-folding hydrogel in vitro model for ductal carcinoma. Tissue Eng Part C Methods. 2016;22:398–407
- Ding, A., Jeon, O., Cleveland, D., et al. Jammed Micro-Flake Hydrogel for Four-Dimensional Living Cell Bioprinting. Advanced Materials. 2022; 34, 2109394.
- Wang, X., Qin, X.-H., Hu, C., et al/ 3D Printed Enzymatically Biodegradable Soft Helical Microswimmers. Advanced Functional Materials, 2018; 28, 180
About The Author:
Tim Sandle, Ph.D., is a pharmaceutical professional with wide experience in microbiology and quality assurance. He is the author of more than 30 books relating to pharmaceuticals, healthcare, and life sciences, as well as over 170 peer-reviewed papers and some 500 technical articles. Sandle has presented at over 200 events and he currently works at Bio Products Laboratory Ltd. (BPL), and he is a visiting professor at the University of Manchester and University College London, as well as a consultant to the pharmaceutical industry. Visit his microbiology website at https://www.pharmamicroresources.com.