Considerations for Development of FRET Assays
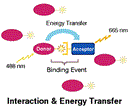
Introduction
FRET assays provide a robust assay platform for High Throughput Screening and have become the assay of choice because of their many benefits, which include:
- Flexible assay format (assays can be quantitative or kinetic, direct or indirect [inhibition or competition]
- Assays can measure protein-protein, protein-nucleic acid, receptor-ligand and immunochemical interactions [immunoassays], nucleic acid hybridization and enzyme activity).
- Homogeneous (just pipette, incubate & measure)
- Rapid, highly sensitive & automatable
- Miniaturizable
- Stable reagents
- Can be conjugated with well-known protein chemistries.
Fluorescence Resonance Energy Transfer (FRET) takes place between two paired dyes when the donor dye absorbs a photon, elevating an electron to a higher energy state and, through resonance, the excitation of this electron is passed to another electron in a second dye, the acceptor. This energy is then re-emitted as a photon that is less energetic, and therefore of a different color (longer wavelength), than the photon initially absorbed. FRET only takes place when dyes are in close proximity (Figure 1).
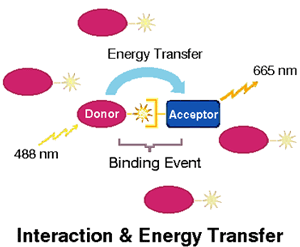
Since FRET can only occur when the participating dyes are very close together, it can be used as an indicator of proximity between pairs of molecules, such as those involved in binding reactions, labeled with pairs of suitable dyes. When proximity based on binding produces FRET, the fluorescence of the mixture of dyes is changed in two ways: the fluorescence of the donor molecule is reduced, since energy which would have been released as photons is instead transferred through resonance to the acceptor, and the fluorescence of the acceptor is increased, due to the release as photons of energy obtained through energy transfer.
Many FRET-based assays involve the measurement of both of these changes, providing an enhanced signal.
FRET assays can be either direct, where dye is attached to a reactant to be assayed, or indirect, where an unknown is tested for its inhibitory or competitive effects on a binding reaction between two known, labeled molecules.
The two major dye classes presently used in FRET are phycobiliproteins and lanthanide derivatives. Phycobiliproteins are water-soluble proteinaceous accessory pigments found in many species of algae (TechNote TNPB100, Phycobiliprotein Overview). For over a decade, they have been widely used in flow cytometry, where they are generally regarded as the brightest commonly available fluorescent tags. Their brightness is achieved due to the presence of multiple fluorophores (individual fluorescent moieties) in each molecule, combined with quantum efficiency among the highest observed for any dye.
In nature, phycobiliproteins participate in FRET in their role as photosynthetic accessory pigments. Energy is passed along a chain of phycobiliproteins from phycoerythrin -> phycocyanin -> allophycocyanin and then on to chlorophyll a. Proximity between phycobiliproteins is maintained in a molecular assemblage called a phycobilisome, which may itself have utility as a fluorescent tag (TechNote PB100.2 Phycobilisomes). Thus, phycobiliproteins have evolved as extremely efficient vehicles for the absorption and transfer of light energy, making them particularly suitable for use in FRET assays (TechNote TNPJ100.2 Phycobiliproteins in FRET Assays).
Due to special properties of lanthanide-based dyes, they are frequently paired with phycobiliproteins in assays referred to as time-resolved FRET (TR-FRET). When sequestered as chelates or cryptates, lanthanides can be used as a FRET donor. The long fluorescence lifetime of these lanthanide complexes is used to avoid certain assay design issues concerning background fluorescence (TechNote TNPJ100.1, FRET vs. TR-FRET). The phycobiliprotein allophycocyanin (APC, sometimes called XL655) is commonly paired with these long-lifetime donors. APC's multiple fluorophores enhance its ability to successfully receive energy transfer, and its emission peak conveniently falls between the multiple emission peaks of the most common TR-FRET donor, europium (Figure 2).
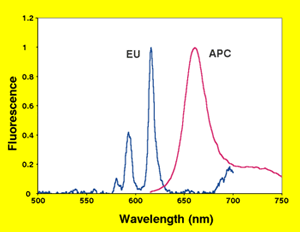
Assay Development
The development of FRET assays requires attention to several aspects of the assay system. For the most part, these same considerations apply whether it is a FRET or a TR-FRET assay under development. See TechNote TNPJ100.10 for an example of assay development for phycobiliprotein FRET.
Selection of the Proximity Pair
The primary function of the donor molecule is as a light antenna, collecting light energy that can be passed along to the acceptor. A donor with a large Stokes' shift is desirable, permitting excitation at a wavelength well below that of acceptor excitation. An acceptor is selected on the basis of its ability to match the vibrational level of the donor fluorophores, as inferred from overlap between the donor emission spectrum and the acceptor excitation spectrum. However due to other factors, such as dipole alignment and distance between molecules in the experimental system, the suitability of a particular proximity pair must always be confirmed empirically. Prediction of suitability for FRET is particularly difficult with phycobiliproteins, where the presence of multiple fluorophores in a single molecule complicates predictions. In some cases, high-efficiency phycobiliprotein FRET can be achieved even with only moderate spectral overlap (TechNote TNPJ100.1 FRET vs. TR-FRET).
Illumination and Detection Windows
Illumination wavelengths for FRET assays should be set based on understanding of the excitation characteristics of both the donor and the acceptor. Calculation of the ratio of donor excitation to acceptor excitation as a function of wavelength can be illuminating in this regard (TechNote TNPJ100.6 Illumination and Detection Windows in FRET Assays). Off-peak excitation of the donor is frequently an acceptable alternative because of the excellent brightness of phycobiliprotein reagents. Selection of detection wavelengths should take into account the analogous comparison of donor and acceptor emission spectra. Self-quenching (see below) should also be taken into account when selecting the detection window.
Self-Quenching
Acceptor dyes with small Stokes' shifts, including the popular dye Allophycocyanin must be added to FRET assays in moderation, due to the potential for significant reabsorption of emitted photons by the dye itself. If the dye is present in significant excess, this can substantially reduce the apparent FRET signal (TechNote TNPJ100.3 Self-quenching in Fret Assays)
Signal, Background and Noise
In any assay, the ultimate objective is to maximize the signal-to-noise ratio of the results. In TR-FRET, one benefit of time-delayed detection is the reduction in background signal due to the acceptor emission that results from off-peak excitation of the acceptor by the illumination source. Since background is reduced, variability in the background is typically also reduced, leading to reduced variability in calculated net signal.
However, signal-to-background and signal-to-noise should not be confused; the benefits of reduction in noise due to low background are obtained at the expense of a weaker signal, due to:
- the poor antenna properties of the lanthanide and
- the restriction of fluorescence detection both in time (due to detection in a limited time window) and in wavelength (due to the narrow window between the multiple emission peaks of lanthanides).
This weaker signal is more subject to variability (noise).
In phycobiliprotein FRET, steps may be taken to reduce background through appropriate selection of dyes and detection windows (see above), but the stronger signal can be more than enough to offset the disadvantages of a somewhat higher background.
Data Collection and Interpretation
In commercially available TR-FRET instruments, acceptor and donor fluorescence are measured in two separate detection windows, frequently referred to as Channel A and Channel B, respectively. Results are typically reported as a ratio of Channel A to Channel B, which amplifies the apparent response signal, since FRET will normally result in both an increase in Channel A and a decrease in Channel B as donor fluorescence is traded off for acceptor fluorescence.
However, investigators are increasingly finding that significant information is lost by examining only the A:B ratio, and that changes in both values should be viewed in concert. The same considerations apply for data analysis in phycobiliprotein FRET (TechNote TNPJ100.8 Data Collection and Interpretation in FRET Assays).
Assay-independent Effects on Signal
Several processes that take place in assay mixtures result in signal changes that may be misinterpreted as assay activity or may mask assay activity:
Quench - When the sample to be assayed contains light-absorbing substances, signal in either channel, or both channels, can be reduced. This serves to offset apparent Channel A response and enhance apparent Channel B response. Examination of the A and B signals, rather than just the ratio of the two, can in many cases permit identification of samples with significant quench.
Autofluorescence - Fluorescence of the sample to be assayed can appear in either channel or both. In TR-FRET, most autofluorescence subsides before measurements are taken. In FRET, autofluorescence effects can be minimized by:
- selection of an acceptor with emission wavelength greater than 600 nm, where autofluorescence is minimal and
- appropriate analysis of the A and B signals.
Non-specific Energy Transfer - Many fluorescent reagents, due either to inherent properties or to properties acquired through conjugation, can bind directly with other fluorescent reagents. Though the affinity of such binding is typically much lower than that observed in assays, the resulting fluorescence can nevertheless be significant relative to assay signal, and is not uncommon with some commercially available TR-FRET reagents. Assay design must include appropriate blanks for reagent interactions and, preferably, use reagents specifically designed to minimize this source of false signal (TechNote TNPJ100.5 Non-specific Energy Transfer in FRET Assays).
For more information: Kim Granger, Prozyme, 1933 Davis Street, Suite 207 San Leandro, CA 94577-1258. Tel: 510-638-6900. Fax: 510-638-6919.
Glossary
Acceptor - In resonant energy transfer, the molecule that receives the energy transfer and emits a photon.
Background - Raw signal when assay activity is zero.
Channel A - Fluorescence measured through the filter chosen to measure acceptor fluorescence; increases when FRET occurs.
Channel B - Fluorescence measured through the filter chosen to measure donor fluorescence; decreases when FRET occurs.
Donor - In resonant energy transfer, the molecule that absorbs a photon and initiates energy transfer to the acceptor.
Fluorescence - Energy emitted as photons when excited molecules return to their ground state.
Fluorophore (chromophore) - The chemical moiety of a dye molecule which absorbs light and, in the case of a fluorophore, emits light through fluorescence.
FRET - Fluorescence Resonance Energy Transfer- In two adjacent dyes, the energy of an excited electron in one dye (the donor) is passed to an electron of an adjacent dye (the acceptor) through resonance, then released as a photon.
HTRF - Homogeneous Time-Resolved Fluorescence, a trademark of Packard Instruments.
Noise - Statistical variability in the response of the assay from all sources, including: variation in actual quantities of reagents added or their concentration, instrument output variability, geometry of plates, uncertainty or variability in background, etc.
Phycobiliproteins - Photosynthetic accessory pigments found in cyanobacteria, red algae, and cryptomonads that absorb light in spectral regions where chlorophyll absorbs poorly and transfers energy to chlorophyll for use in photosynthesis. Chromophores (linear tetrapyrroles called bilins) are attached to a protein framework.
Proximity pair - Two fluorescent dyes selected for their ability to successfully achieve FRET when brought into close proximity in a FRET Assay or in a tandem dye.
Quantum efficiency - Proportion of absorbed photons released as fluorescence.
Quench - Absorption of light in an assay mixture by either reactants or impurities.
Raw signal - Signal before correction for background.
Self-quenching - Tendency of fluorophores with small Stokes shifts to absorb photons at some of the same wavelengths at which they emit.
Signal - The "real" response of an assay to activity in the sample, independent of all sources of error and statistical variability, corrected for any background.
Stokes' shift - Difference in wavelength between the fluorescence emission and excitation peaks of a given dye.
Tandem fluorescent dye (tandem dye, tandem conjugate) - Two fluorescent dyes capable of FRET covalently coupled. Tandem dyes are used when increased Stokes shifts or multiple emission wavelengths from a single excitation wavelength are desired.